RESEARCH PROJECTS
Research Projects (April 2014)
-
Investigation of mechanisms regulating neural stem/progenitor cell fate during brain development and in the adult brain
- Roles of Polycomb group proteins and other chromatin modifiers in neural stem/progenitor cell fate during neocortical development
- Signaling pathways that regulate the fate commitment and differentiation of neural stem/progenitor cells
- Mechanisms that regulate the generation and maintenance of adult neural stem cells
- Investigation of chromatin-level regulations of neuronal differentiation/maturation and activation
- Dissection of signaling pathways regulating cell migration and metastasis
- Dissection of signaling pathways regulating cell death and innate immunity
- Roles of Polycomb group proteins and other chromatin modifiers in neural stem/progenitor cell fate during neocortical development
- Signaling pathways that regulate the fate commitment and differentiation of neural stem/progenitor cells
- Mechanisms that regulate the generation and maintenance of adult neural stem cells
1. Regulation of neural stem/progenitor cell fate
during mouse neocortical development
![]() |
(Red,Brn2; Green,Tbr1; Blue,Hoechst) |
We are currently tackling fundamental questions in neurobiology and stem cell biology including- what defines the ‘stemness’ or ‘differentiation potential’ of NSCs?; What mechanisms govern the temporal fate switch of NSCs during neocortical development? (what is the timer?) ; which embryonic cells are the origin of adult NSCs and what are special about these cells?; what regulates the diversity of neuronal and astrocytic subtypes, and what coordinates their area-specific organization? Through answering them, we would like to unveil basic mechanisms of brain development and to obtain knowledge useful for regenerative medicine.
- Epigenetic control of neural stem/progenitor cell fate
Tissue stem cells in the developing central nervous system (CNS), called neural stem cells (NSCs) or neural precursor/progenitor cells (NPCs), produce various neuronal and glial cell types in a developmental stage-dependent manner. The temporal and spatial regulation of NSCs’ differentiation potential is key for precise formation of the complex structure of the CNS.
Throughout development, the differentiation potential changes as NSCs pass through phases of expansion, neurogenesis, and astrogenesis. Interestingly, both cell intrinsic and cell extrinsic mechanisms appear to be important for these transitions. We are interested in examining the mechanisms underlying the timing of these transitions. The timing of the transition between the neurogenic and astrogenic phases is critical for brain development, in particular because the length of the neurogenic phase is an important parameter in determining the number of neurons in each brain region. We found that the polycomb group complex (PcG) restricts neurogenic competence of NPCs and promotes the transition of NPC fate from neurogenic to astrogenic.
Moreover, PcG represses the promoter of the proneural gene neurogenin1 and 2 in a developmental stage-dependent manner and serves as a developmental timer (Hirabayashi et al. Neuron 2009; Hirabayashi and Gotoh, Nat. Rev. Neurosci. 2010). We found that PcG also plays an essential role in regulating NPCs’ fate transitions within the neurogenic phase, which occur as the switch from producing one neuronal subtype to another (Suzki-Morimoto et al. unpublished). We are now investigating the mechanism by which PcG acts in a local- and developmental time-dependent manner. As this is a common and important issue for the fate control of tissue stem cells, we hope that our work will shed light on general mechanisms of stem cell fate control.
Previous studies on chromatin regulation have mainly focused on specific genomic loci. Surprisingly, we found that, in addition to “local” changes in the chromatin state at specific loci, the “global” chromatin state in the whole genome changes during neural development (Kishi et al., Nat. Neurosci. 2012). This work revealed that HMGA proteins mediate the open chromatin state in early-stage NSCs, conferring on them the neurogenic potential (Kishi et al. Nat. Neurosci. 2012).
- Signaling pathways that regulate neural stem/progenitor cell differentiation
We have been investigating signaling pathways that control the NSC fate and differentiation. We found that the transcription factor Stat3 maintains the NSC pool (Yoshimatsu et al. Development 2006) and uncovered that there is crosstalk between Stat3 and the Notch effectors Hes1/5, playing an essential role in maintenance of NSCs (Kamakura et al. Nat. Cell Biol. 2004). We also found that Wnt/beta-catenin signaling instructs neuronal differentiation of NSCs (Hirabayashi et al. Development 2004) and promotes production of neuronal progenitors in the neocortex (Kuwahara et al. Development 2010), whereas PDK1-Akt signaling promotes GABAergic neuronal production in the ganglionic eminences (Oishi et al. PNAS 2009).
In the developing neocortex, once NSCs commit to becoming neurons, they stop dividing and start to migrate toward the pial surface. Thus, we asked what mechanisms couple neuronal fate commitment, cell cycle arrest, and neuronal migration. First, we found that the cdk inhibitors p57 and p27 contribute to coupling cell cycle arrest and neuronal migration (Itoh et al. J. Biol. Chem. 2007). Furthermore, our works indicates that Scratch1 and Scratch2 are the missing link that couples neuronal fate commitment and the onset of migration (Itoh et al. Nat. Neurosci. 2013)- proneural transcription factors, which promote neuronal fate commitment, also induce the expression of Scratch proteins, which in turn trigger the delamination of newborn neurons from the ventricular surface by suppressing the expression of E-cadherin and other proteins essential for cell adhesion. Since Scratch proteins belong to the Snail superfamily that mediates the epithelial-to-mesenchymal transition (EMT) in various contexts, onset of neuronal migration can be considered an EMT-like process.
During the neurogenic phase of mammalian brain development, only a subpopulation of NSCs differentiates into neurons. Our results support the notion that different levels of Dll1 expression among NPCs works through cell-cell interactions, most likely through the Notch-Delta lateral inhibitory signaling pathway, to mediate the selection of differentiating cells (Kawaguchi et al. Development 2008). Dll1 is an essential feedback signal from differentiating cells that suppresses excess neuronal differentiation and maintains the NSC pool both in the embryonic and adult brain (Kawaguchi et al. Nat. Comm. 2013).
- Mechanisms that regulate the generation and maintenance of adult NSCs
![]() |
In contrast to the embryonic NSCs that quickly and sequentially produce a variety of neural cell types in a limited time, adult NSCs have a very different mission. Namely, they have to produce the same sets of neural cell types for a very long time (a lifetime) with little changes in their differentiation potentials. We are very much interested in the differences between embryonic and adult NSCs and in trying to find the molecular basis of these differences.
One prominent feature of adult NSCs is their low frequency of cell division, just like many other adult tissue stem cells. To elucidate the role of the slow cell cycle/infrequent division in adult NSC biology, we identified a cell cycle inhibitor responsible for their slow cell cycle. This inhibitor, the cdk inhibitor p57, is highly expressed in adult NSCs and is reduced upon neurogenic stimuli such as running and kainic acid injection, resulting in the release from the cell cycle arrest. Importantly, while a short-term deletion of p57 increases adult neurogenesis, a long-term deletion of p57 decreases the number of adult NSCs and neurogenesis. This indicates that the slow cell cycle of adult NSCs is essential for preventing the exhaustion of these cells.
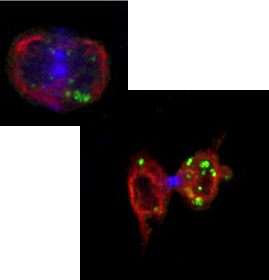
Adult NSCs are supported and controlled by a microenvironment (niche), which is essential for their maintenance. We found that the Notch ligand, Dll1, is a critical part of this niche and is essential for NSC maintenance. Dll1 is expressed in “activated” NSCs and, surprisingly, segregated asymmetrically to one daughter cell during mitosis. Dll1-inheriting cells then commit to the neuronal fate and send the signal for NSC maintenance to the other daughter cell, resulting in asymmetric cell fate between the two daughter cells of an NSC. This study thus unveils that the sister cell is an important “niche” for NSC maintenance. The asymmetric segregation of Dll1 is likely important in maintaining the NSC pool size through divisions.
Top ↑
3. Regulation of cell migration and invasion
by the proto-oncogene Akt
![]() |
Akt is a serine/threonine kinase whose excess activation is often associated with malignant cancers. Akt has been suggested to promote tumorigenesis via aberrant promotion of cell survival, proliferation, transloation/metabolism etc. We identified several targets of Akt in the context of cell survival such as Mdm2, a ubiquitin ligase for the tumor suppressor p53. Akt-mediated phosphorylation of Mdm2 results in ubiquitination and degradation of p53 (Ogawara et al. J. Biol. Chem. 2001). Since p53 is responsible for DNA damage-induced apoptosis, Akt activation makes cells resistant to DNA damage. We also found that Akt plays prerequisite roles in growth factor-stimulated directional migration of fibroblasts, which may be involved in tumor cell invasion during metastasis (Higuchi et al. Curr. Biol. 2002).
We have also investigated the mechanisms by which growth factors regulate Akt signaling.
We found that PAK serves as a scaffold and regulates the efficiency, localization and specificity of the PDK1-Akt pathway
(Higuchi et al. Nat. Cell Biol. 2008). PAK mediates Akt-stimulated cell migration but not survival.
Akt promotes cell migration partly through microtubule stabilization (Onishi et al. Genes Cells 2007) and FAK-dependent adhesion turnover (Higuchi et al. JCS 2013).
[This project will be led by Dr. Maiko Higuchi from April 2014]
Top ↑
4. Roles of stress-activated MAP kinases in cell death
and innate immunity
Previously, we identified MAP kinase and its activator (MAPKK) in growth factor-stimulated mammalian cells as well as in Xenopus oocytes. A subgroup of our lab is continuing the study on MAPK signaling, now focusing on a member of the stress-activated MAPK superfamily, JNK.
JNK plays a pivotal role in cell death induced by a variety of cellular stresses (cf. Ura et al. PNAS 2001). Several proapoptotic proteins, including Bad and FOXO3a, commonly become associated with 14-3-3 upon phosphorylation by survival-mediating kinases such as Akt and localized in the cytoplasm. We found that phosphorylation of 14-3-3 by JNK releases Bad and FOXO3a from 14-3-3 and promotes their mitochondrial translocation, allowing them to induce apoptosis, thereby antagonizing the effects of Akt signaling (Tsuruta et al. EMBO J. 2004; Sunayama et al. J. Cell Biol. 2005).
![]() |
(Red,Active Caspase-3; Green,Infectedcells; Blue,Hoechst) |
We found that JNK is also responsible for the enhancement of Ca2+-evoked release of secretory vesicles by NGF treatment in PC12 cells. JNK directly associates with and phosphorylates synaptotagmin-4, causing it to become localized to mature secretory vesicles, and enhancement of their Ca2+-evoked release. These results have revealed a previously unrecognized molecular link between peptidyl growth factors and secretory machinery (Mori et al. EMBO J. 2008).
Currently, we are investigating the roles of stress-activated MAPK pathways in innate immunity. At early stage of viral infection, host cells employ two strategies to prevent viral expansion: interferon (IFN) response and apoptosis. It is, however, unknown what conditions might discriminate between the usage of these two strategies and what molecular mechanisms would underlie such discrimination. We are now working on identifying the mechanisms that mediate the choice between these two host defense strategies (Okazaki et al., unpublished).